Contents
What Are the Basics of FDM, SLA, and SLS Technologies?
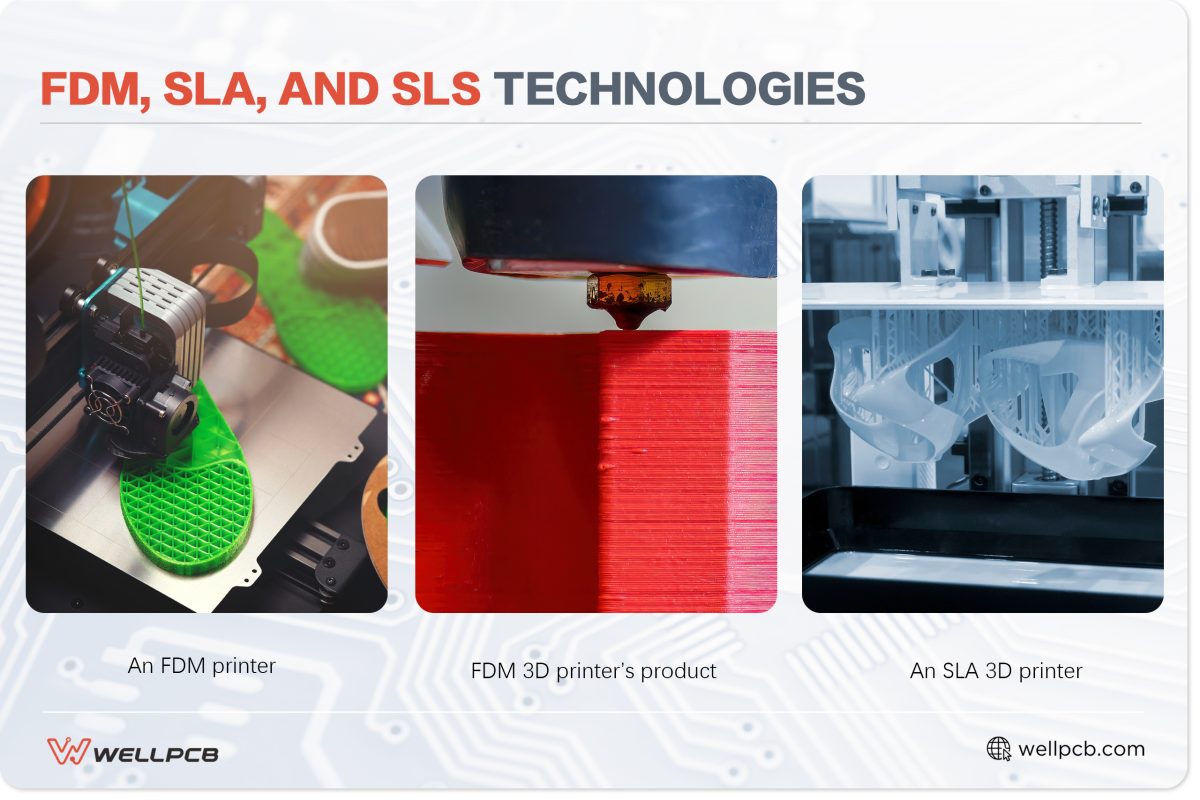
How Does FDM (Fused Deposition Modeling) Work?
Fused Deposition Modeling (FDM), also known as Fused Filament Fabrication (FFF), is the most widely used 3D printing technology due to its affordability and extensive material options.
The process involves melting a plastic filament, which is deposited layer by layer on a substrate according to the 3D design of the final product. This filament is fed from spools into the printer’s heated nozzle, which precisely melts and places it onto the print bed until the object is complete.
A key challenge of FDM is that this layer-by-layer deposition often creates bumpy surfaces on vertical planes. Additionally, FDM printers struggle with complex geometries like overhangs, undercuts, and hollow parts, often requiring supporting structures during printing.
FDM is ideal for developing quick proof-of-concept models or form-and-fit prototypes in business settings. When using advanced materials like carbon-fiber-reinforced plastic, FDM printers can produce more durable products. As such, the choice of material is key in determining the quality of the final product.
Advantages of FDM 3D Printing
- Affordable hardware
- Works with multiple types of plastic and composite materials
- Non-toxic, although some materials like ABS, nylon, and ASA may produce toxic fumes
- The cost of equipment and set-up is relatively low
Disadvantages of FDM 3D Printing
- Limited design options, as it struggles with acute angles, thin walls, and sharp edges on vertical planes
- Often forms bumps or stair-steps on vertical planes
- Relatively inaccurate, with tolerances of between 0.1mm and 0.25mm
- Maintaining a consistent temperature in the building chamber can be challenging
- Often requires significant finishing work to smooth surfaces and refine details
What Is SLA (Stereolithography) and How Does It Operate?
Stereolithography (SLA), often referred to as resin 3D printing, creates 3D objects using resin. While it was the first additive manufacturing technology, SLA hasn’t seen as widespread adoption as FDM due to its higher cost and complexity.
SLA involves using a light source to cure or harden liquid resin in a process known as photopolymerization, building the object layer by layer to form a rigid structure. While traditional SLA uses a laser beam as the light source, emerging technologies like DLP use digital light projectors, whereas MSLA (Masked SLA) uses LEDs.
SLA is known for creating objects with smooth surface finishes, higher dimensional accuracies, and tight tolerances. As a result, it’s ideal for creating end-use products, tooling, and functional prototypes.
The quality of the final product will ultimately depend on the type of resin used, with various formulated resins available that offer different optical, mechanical, and thermal properties.
SLA is extremely versatile and can be used to 3D print products for a broad range of applications, including aerospace, automotive, healthcare, and dentistry.
Advantages of SLA 3D Printing
- Produces products with smooth surface finishes
- Capable of printing thin parts (0.05–0.15mm), with layer thickness varying by resin
- Finished parts can be painted, enhancing their aesthetic and functional properties.
- Economical for low-volume production (up to 20 parts)
- Prints quickly
Disadvantages of SLA 3D Printing
- High cost of resins and printers
- Requires labor-intensive cleaning and curing
- Resins and their fumes can be toxic
- Waste material is not recyclable
- Lasers need periodic recalibration to ensure accuracy
How Does SLS (Selective Laser Sintering) Work and What Are Its Benefits?
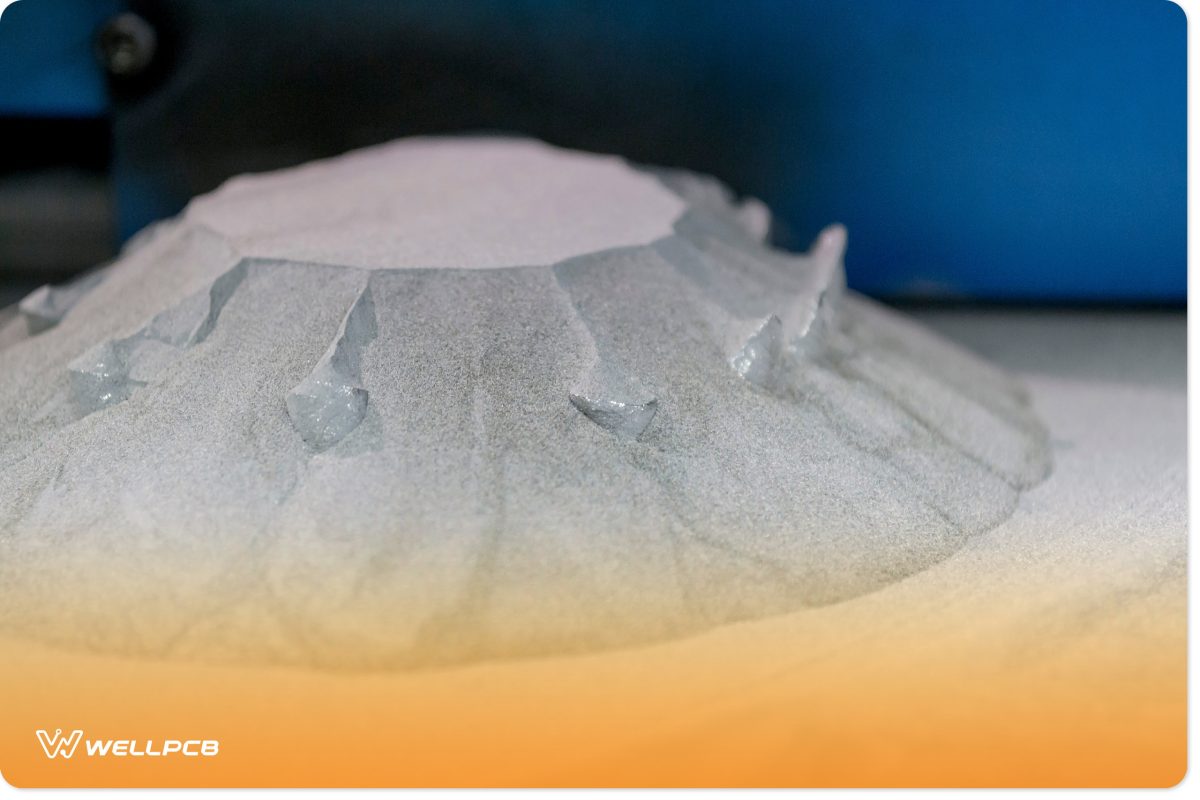
Selective Laser Sintering (SLS) is the most common additive manufacturing technology for industrial applications, known for its reliability and ability to produce strong, functional end-use parts.
This 3D printing process uses a laser to sinter powdered metal—essentially heating it without melting—to fuse particles.
This occurs in a sealed chamber filled with inert gas to prevent oxidation, which is preheated to a temperature just below the powder’s melting point.
The laser then selectively provides the additional energy needed to reach the melting point, according to the design.
This process repeats layer by layer; when sintering is complete in one layer, a re-coater replenishes the powder to form another layer.
The unsintered powder surrounding the object provides the necessary support during the printing process.
SLS can print with materials like engineering thermoplastics (notably nylon 11 and 12), wax, and elastomers.
It’s highly productive and offers a cost-efficient solution for both functional prototypes and final products, especially in low-volume manufacturing, where it serves as a low-cost alternative to injection molding.
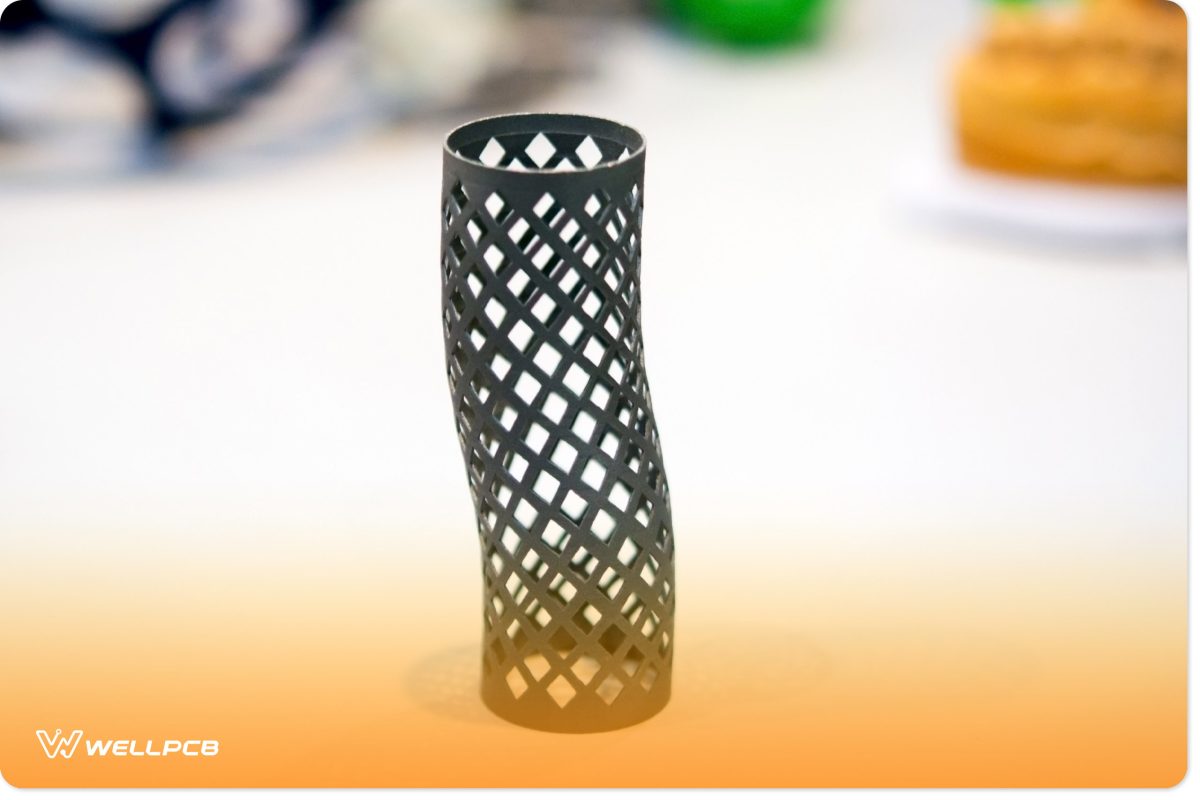
Advantages of SLS 3D Printing
- Does not require support structures
- Surplus powder can be reused, reducing waste
- Can create complex geometries with movable internal and external parts
- Produces products with smooth surfaces that require minimal post-processing
Disadvantages of SLS 3D Printing
- Industrial-grade SLS 3D printers are expensive
- Removing excess powder can be messy and time-consuming
- Printing, especially large objects, takes a long time
- Chambers must be cleaned meticulously when changing materials to prevent contamination
What Are the Key Factors When Comparing FDM, SLA, and SLS?
When choosing between these 3D printing technologies, the most important factors to consider are materials, cost, efficiency, and surface finishing. Let’s examine each of these in detail.
Materials
FDM | SLA | SLS |
Standard thermoplastics, such as: PLA ABS Nylon PETG ASA | Various formulated resins, including: Standard PU High temperature Flexible Medical and dental Silicone Ceramic Flame retardant Clear Draft Rigid | Engineering thermoplastics, including: Nylon 12 Nylon 11 Composites, such as: Carbon-fiber-reinforced thermoplastics TP UKevlar Fiberglass Carbomorph Polypropylene and nylon composites |
- Standard thermoplastics are relatively cheap but don’t form smooth, sturdy, or durable products. This is largely due to the layering process inherent to FDM printing.
- Resins, on the other hand, form products with smooth surfaces and tight tolerances, which can be costly.
- The engineering plastics and composites used in SLS 3D printing form the most durable products of the three, coupled with a consistently high-quality finish. However, these materials are more expensive as a result.
Cost
Cost Factor | FDM | SLA | SLS |
Initial Setup | Budget FDM printers and starter kits: from $200 Professional FDM printers (desktop): $2,000–$8,000 Industrial FDM printers: from $15,000 | Budget resin printers: $200–$1,000 Professional resin printers: $2,000–$10,000 Large-format resin 3D printers: $8,000–$25,000 | Benchtop industrial powder 3D printers cost around $30,000 but require cleaning and powder management stations that collectively cost around $60,000 Traditional industrial SLS powder printers cost around $200,000 |
Material Costs | $50–$150 per kilo for standard filaments $100–$200 per kilo for engineering filaments $100–$200 per kilo for supporting materials | $100–$200 per liter for standard and engineering resins $200–$500 per liter for biocompatible resins | $100 per kilo for nylon 12 and 11 |
Training | Requires minor training for build setup, machine operation, and product finishing. Moderate training is needed for machine maintenance. | Almost plug-and-play, but requires minor training on setup, production, finishing, and machine maintenance. | Moderate training is needed for build setup, machine operation and maintenance, and product finishing. |
Production Speed | Quick printing time for low volumes, but products might need extensive post-processing to smoothen the surfaces. | Quick printing time, but needs additional time for post-processing to clean and remove marks left by supporting structures. | Takes a long time to print and requires supporting powder removal, with additional time needed for post-processing. |
3D printing using FDM is the most affordable option, but it limits your capabilities. As such, its low-cost production is best suited for simple prototypes or products like toys.
Speed and Efficiency
When producing a small batch of assemblies with minimal parts, SLA 3D printing is the fastest option—especially when considering emerging technologies like MSLA and DLP. FDM ranks second, as the process of moving the extruder across the bed to deposit molten plastic is quicker than the sintering required by SLS, which also requires significant cooling time.
However, this dynamic changes when producing larger volumes of assemblies with numerous parts. SLS excels in this scenario as the laser can be directed at different parts of the powder layer quickly, significantly increasing throughput and producing these assemblies in one go. The only downside remains the cooling time after printing. As such, SLA is still the fastest option as it doesn’t require any cooling time.
FDM has the slowest throughput for large volumes as the nozzle has to follow an intricate pattern to lay out the molten plastic, which takes significant time for each layer.
Here’s a time comparison between FDM, SLA, and SLS printers when producing electrical connectors with layer resolutions of 120 microns for FDM, 100 microns for SLA, and 110 microns for SLS.
Assembly/Parts | FDM | SLA | SLS |
1 assembly with 2 parts | 2.6 hours | 1 hour | 3.5 hours + 6.5 hours of cooling time |
50 assemblies with 100 parts (2 each) | 250 hours | 13 hours | 13 hours + 13.8 hours of cooling time |
Surface Finish and Detail
FDM operates like a hot glue gun, laying molten plastic in layers that often leave noticeable lines. These require extensive post-processing to smoothen. The resulting products also lack true transparency or translucency due to light refraction from these bumpy surfaces.
On the other hand, SLA printers excel in creating parts with smooth finishes that rival injection molding. The lack of layer lines means true transparency or translucency is achievable with appropriate materials. This makes it ideal for 3D printing medical and dental products, such as dentures, which require naturally smooth surfaces that accurately replicate gum and enamel textures.
Although SLS-printed components initially have a rough, grainy texture, they emerge with a uniform surface finish that can be sandblasted and polished to create smooth, glossy surfaces. And unlike FDM and SLA, SLS does not require the removal of marks left by support structures.
Which Technology Is Best for Your Industry?
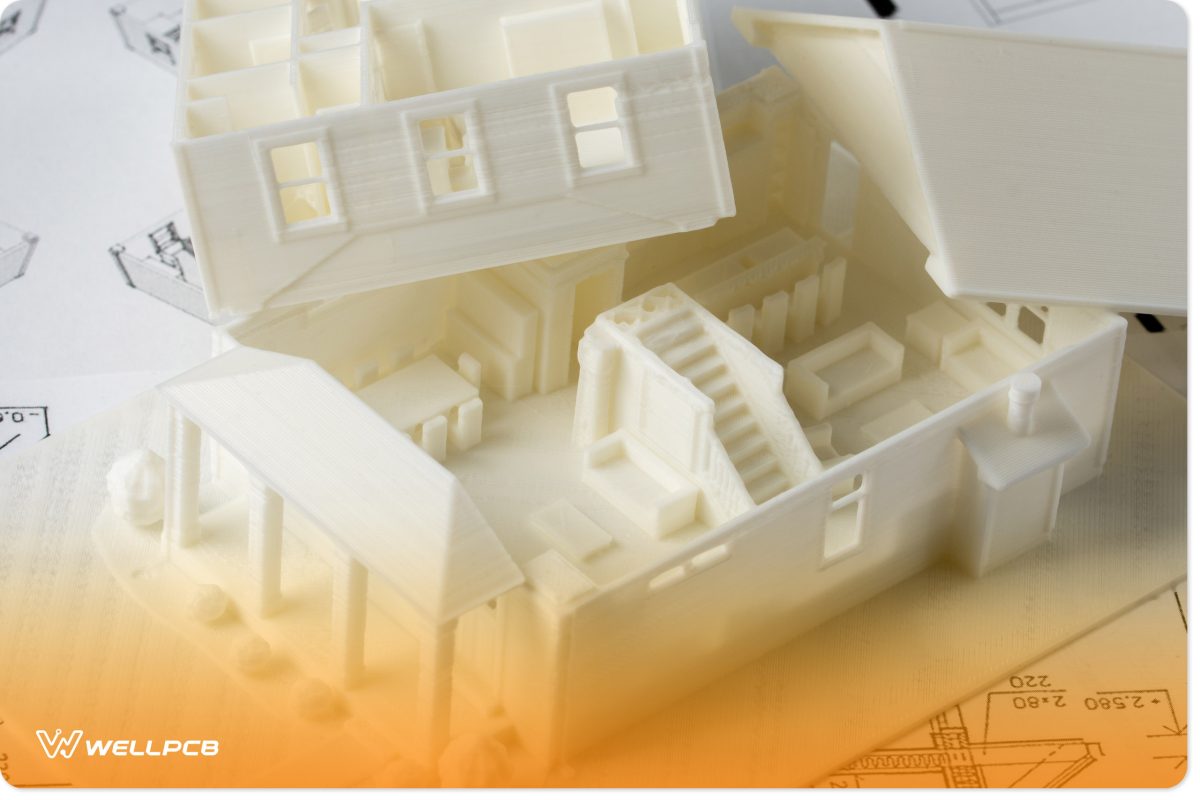
What 3D Printing Technology Is Best for Manufacturing?
Engineering and manufacturing usually rely on thermoplastics because they are more durable than resin and more resistant to environmental factors. As such, FDM and SLS are the most suitable technologies for those in the manufacturing industry.
While FDM typically creates mechanically weak components, industrial FDM printers can produce sturdy plastic products such as electronic housings, selective brackets, and wing mirrors for cars. Industrial-grade FDM can also be used to make motorcycle helmets, jigs, fixtures, and components for aerospace and aviation applications.
Why Is SLA the Best Choice for Healthcare?
SLA or resin printing is ideal for healthcare applications due to its high resolution, precision, and dimensional accuracy, coupled with its smooth surface finish. As it lacks layer lines, SLA is the most suitable technology for 3D printing medical and dental models. That mimics the smoothness of human bones and teeth enamel.
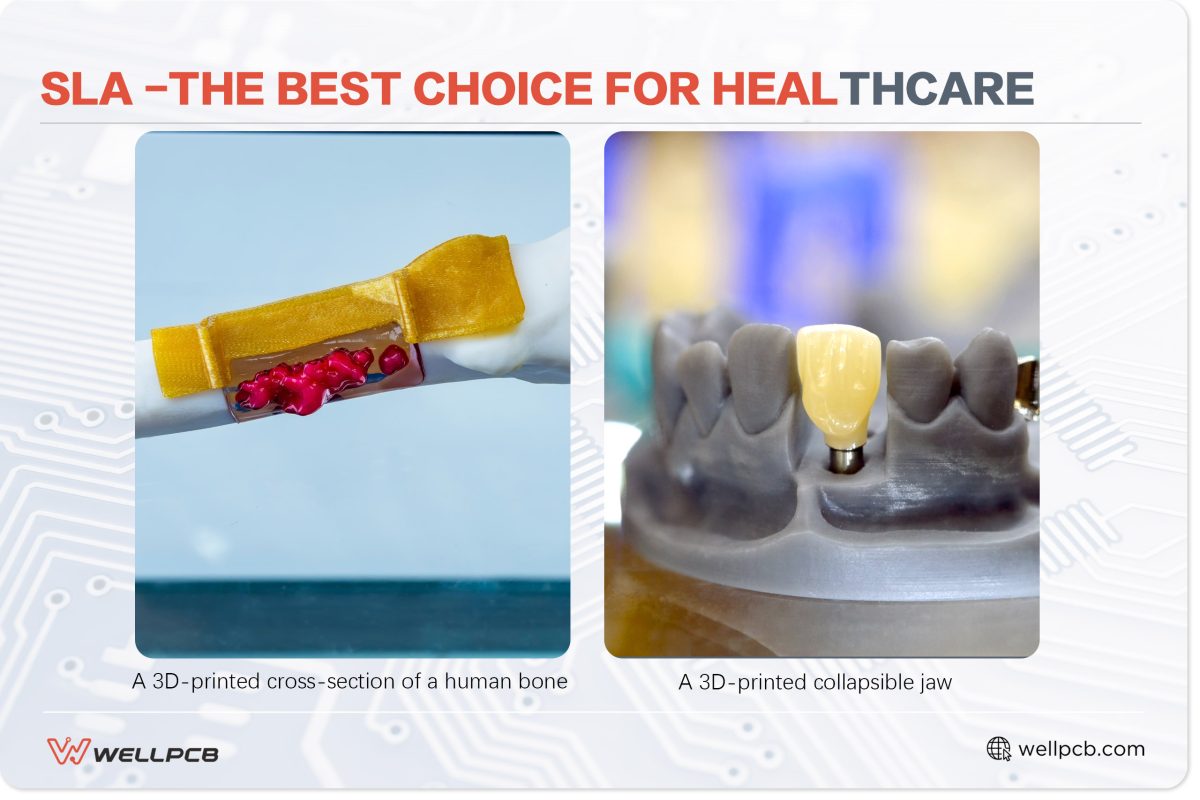
Biocompatible resins like MED610 can be used to 3D print medically approved surgical guides that are rigid enough to hold surgical instruments.
Resins can also be used to 3D print custom fixtures, jigs, and tools. As an industry example, Pankl Racing Systems leverages resin printing to significantly reduce costs and lead times.
Why Are SLS and FDM Ideal for Automotive and Aerospace?
Industrial FDM printers can produce non-critical aerospace and aviation parts. The Airbus A350 is a prime example, as it has over 1,000 FDM printed flight components. According to Airbus, this method reduces production time and costs.
3D printing automotive parts can also make cars lighter and create more opportunities for customized plastic sections like dashboards.
But SLS leads the way in aerospace and automotive parts manufacturing, primarily due to its micron-level precision and impressive strength. For instance, turbine blades and wing ribs can be 3D printed using reinforced plastics. Other SLS-printable components include seat frames, fuel nozzles, brackets, and propulsion systems, often as prototypes before full-scale production.
Aerospace and aviation components must typically be lightweight to escape the earth’s gravity and optimize fuel efficiency. Airbus recently approved a high-performance, flame-retardant polyamide for SLS 3D printing with excellent long-term stability and chemical resistance. The company plans to use this material to make non-critical and low-critical parts, like interior cabin fittings.
Future Trends in FDM, SLA, and SLS Technologies
What Are the Emerging Developments in FDM, SLA, and SLS?
FDM
Key emerging developments in FDM include innovative new materials, improved tracking and precision through closed-loop feedback systems, automatic bed clearing, more advanced toolpaths, additional color options, and multi-material printing.
The trend towards new materials is especially promising, as FDM is not constrained to UV or light curing. Therefore, materials to look out for include electrically conductive plastics, magnetic, carbon-fiber infused, metal-filled, woodfill, and corkfill plastics, as well as other exotic materials.
Researchers are also looking at ways to include multiple materials in a single filament, each contributing unique properties. This will enable precise control over the composition and distribution when printing.
SLA
As SLA is limited by UV curing, improvements primarily focus on speed enhancements. Recent advancements in this field include DLP (Digital Light Processing) and MSLA (Masked Stereolithography).
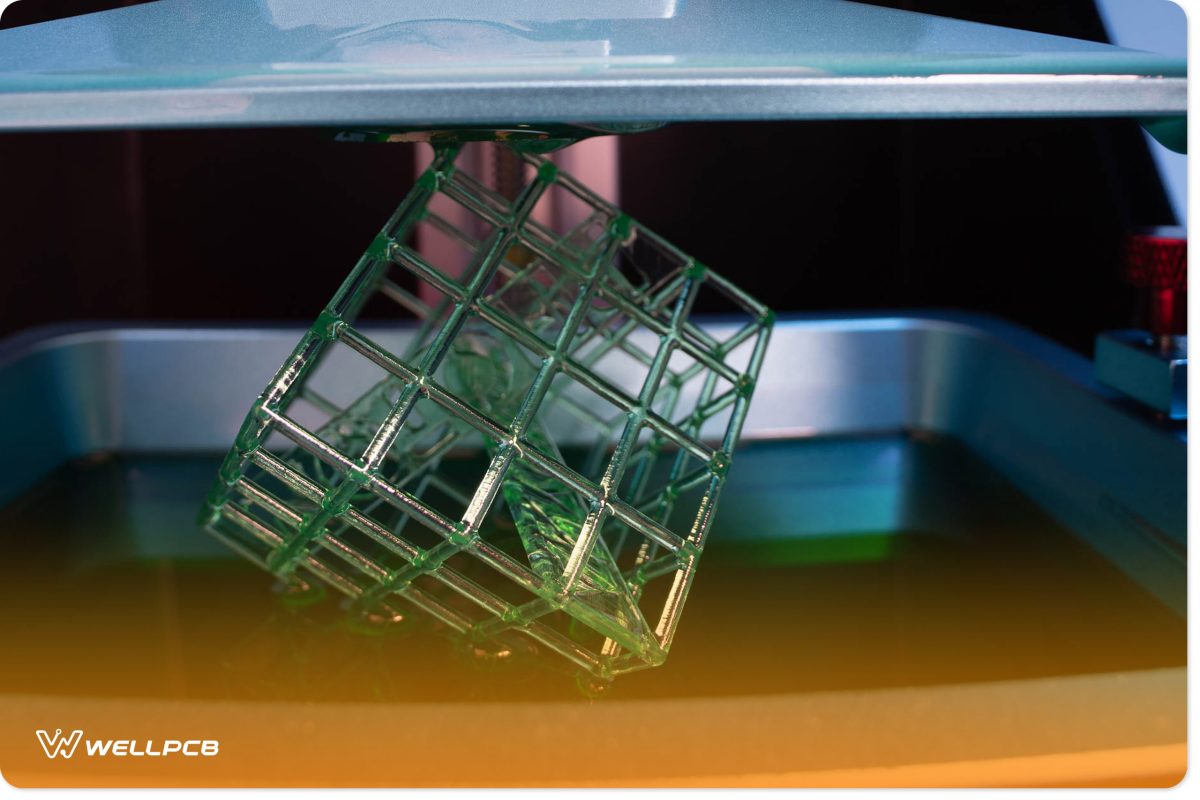
DLP uses a single light source, typically LEDs, to project images onto resin. This projection is achieved through a Digital Micromirror Device (DMD) chip. The LEDs have wavelengths between 385nm and 405nm, which are ideal for curing resin. However, a limitation of this technology is that the printer’s resolution caps at about 4K.
MSLA bumps this resolution to 8K, as it uses an LCD screen with multiple LEDs spread across the build plate. While slight loss occurs as light travels from the screen to the resin, this is usually negligible.
Other recent SLA innovations include Continuous Liquid Interface Production (CLIP) and Two-Photon Polymerization (2PP).
SLS
As SLS produces high-quality parts, emerging developments are pushing it deeper into final or end-use part production. These include the use of dual lasers to boost printing speed and the creation of larger machines to accommodate bigger build volumes, addressing the demand for lightweight, consolidated assemblies.
Additionally, expanding material options and techniques like vapor smoothing streamline production and reduce the need for post-processing.
Researchers are also exploring new applications and geometries using SLS, including:
- Periodic cellular structures
- Dissolvable scaffolding (to support soft and suspended 3D printing)
- Metamaterials for complex geometries with unique properties like negative stiffness
- Biocompatible polymers to print human tissues, such as bones
How Can Businesses Stay Ahead of Technological Changes?
To stay ahead of the curve and remain competitive, businesses should engage with the latest developments in 3D printing.
Stay updated with industry forums, company pages, or the WellPCB blog. The 3D printing section of the blog explores all the latest advances and trends in 3D printing, which we publish as soon as they’re announced.
Conclusion
The key differences between FDM, SLA, and SLM revolve around the materials used: standard thermoplastics for FDM, resins for SLA, and engineering thermoplastics or composites for SLM. While factors like cost, printing speed, and surface finish are also important, your choice of material will largely dictate the appropriate technology.
- For resins: SLA is the preferred technology, excelling in creating high-detail and smooth-finish models.
- For thermoplastics: Both FDM and SLS are viable options, with the choice depending on the specific properties and applications needed.
So first consult your specific use case, then consider other factors to identify the best-fit technology to employ.
If this selection process is challenging, we’d be happy to help. Contact us for a free consultation, where we can discuss your business idea along with any other relevant details. We’ll help get your 3D-printed products ready in no time!